Polylactic acid
Polylactic acid | ||
---|---|---|
Home | Research & Development | Bill of Materials | Manufacturing Instructions | User's Manual | User Reviews | 78px |
Polylactic acid is a thermoplastic suitable for light weight applications and plastic extrusion by 3D printers. Polylactic acid is a bio-degradable polyester polymer of lactic acid. Lactic acid can be formed by fermentation of sugars by microorganisms or synthetically from petroleum. Lactic acid is polymerized via sequential condensation reactions with a catalyst between 130-160 C. Polylactic acid is currently in first generation large scale production using corn starch as a feedstock.
An agroecological approach is proposed to use open source technology to produce lactic acid from a variety of agricultural feedstocks, purify the lactic acid with import replaceable membranes, and polymerize the product with minimal costly catalyst. Initial feedstocks will be converted to input lactic acid by microorganismal fermentation. Bacillus coagulans is proposed as a LA bacterial strain for its thermophilicity, homolactic capabilities, high productivity, and ability to use pentose sugars. A fermentation chamber is in the research and development phase and will be used to conduct batch-fed or continuous fermentation. Membrane separation and purification appears to be an efficient method on a small scale and will be used for initial test runs. Lactic acid will be polymerized in a reaction chamber capable of high temperatures and low pressures. The goal of the first production run is to produce a polymer, characterize its properties, and test it in 3D printing extruders.
Status Brief
Documentation Brief
A review of publicly available information is located at Polylactic_acid/Research_Development. Review of the polymerization step is considered complete, while extensive research on production and purification of lactic acid substrate is still needed. Further research into preparing the product for use in extrusion is still needed.
Current Challenge
Current challenges include the design of an OSE reactor, process for local production of lactic acid, and import replacement of catalysts. A subject matter expert must review the process and offer feedback and critiques.
Background
Feedstock starting materials
A variety of feedstocks can be used as a source of sugar for fermenting microorganisms including high starch plants, high sugar plants, or dairy. Feedstocks should be chosen based upon a high yield of sugars suitable for Lactic Acid Bacteria to use as a reducing agent in fermentation. Please see the page on silage, which could be a source of abundant lactic acid, while also serving as an excellent mechanism for processing biomass into animal food and biogas feedstock.
Lactic acid producing microorganisms
Organisms are chosen with the metabolic capabilities to utilize sugars in the feedstock. Homofermentative species that produce optically pure L(+)-lactic acid as a sole product should be used for maximum yield and to reduce complicating products. With the recent interest in lactic acid as a platform for bioplastics a great deal of research has gone into identifying optimal strains and defining their growth conditions and capabilities. Lactobacillus (bacteria) and Rhizopus oryzae (fungus) have been been identified as platforms for commercial lactic acid production. However, recently (2009) thermophilic Bacillus coagulans (2-6) has been identified as an even more promising microorganism for fermentative lactic acid acid production. Its optimal growth temperature of 50 C is higher than other fermentative species preventing contamination even with unsterilized feedstocks, it produces optically pure L(+) and lacks a D-LA dehydrogenase, and achieves very high conversion efficiency of 97.5%.
There are two main hexose fermentation pathways that are used to classify LAB genera. Under conditions of excess glucose and limited oxygen, homolactic LAB catabolize one mole of glucose in the Embden-Meyerhof-Parnas (EMP) pathway to yield two moles of pyruvate. Intracellular redox balance is maintained through the oxidation of NADH, concomitant with pyruvate reduction to lactic acid. This process yields two moles ATP per mole of glucose consumed. Representative homolactic LAB genera include Lactococcus, Enterococcus, Streptococcus,Pediococcus, and group I lactobacilli. Fermentation is inhibited by endproducts and advances in fermentation technique includes methods to remove lactic acid as it forms allowing continuous operation of bioreactors and higher conversion rates.
Strains could either be isolated from a fermented product or ordered from a supplier. A relatively simple procedure for isolating and identifying LAB from an enriched media can be found here[1]. Alternatively a strain may also be found by contacting relevant research laboratories. Most strains are available for sale under s for-profit license from the American Type Culture Collection (ATCC). In industry Lactobacillus delbrueckii, L. amylophilus, L. bulgaricus and L. leichmanii are reportedly used, as well as mutant strains of Aspergillus niger fungus. Strain selection is a large variable in the process and feedstock and growth parameters must be considered.
Culturing and growing microorganisms
Culturing lactic acid producing microorganisms has been a human endeavor for thousands of years for food preservation, however there are differences in the characteristics of strains optimal for food preservation versus utilitarian lactic acid production. A food preservation LA microorganism must be a nonpathogenic and for maximum benefit should be probiotic, their growth should be slow to maintain the food for an extended period, relatedly growth should be possible at lower temperatures to extend preservation further, full utilization of sugars may not be desirable to maintain a higher nutrient density for human consumption, and a mixture of products may be desired to produce complex flavors. In contrast utilitarian LA microorganisms should grow fast to minimize the process time and decrease the chances of contamination, they should have a high yield and convert as much of the feedstock to the product as possible.
Lactic acid microorganisms can be grown in the OSE fermentor, airtight containers, or as silage. Lactobacilli are optimal for silage and inoculation schemes could be useful. Operation of a fermentor for lactic acid production would be aided by control of pH, removal of product, and features for continuous operation.
High yield lactic acid fermentation is through optimization of the microorganisms environment. The pH of the media will be lowered by the production of lactic acid and maintaining pH in the range of 5-6 is essential to culture health and maximum yield. It is through the production of acid that the organisms gain energy and maintaining favorable redox conditions allow the cells to continue to harvest energy through fermentation. To maintain pH developed techniques were to add calcium stearate or other salts, which also precipitated the salt form of lactic acid for collection, however an equal amount of waste is produced alongside the purified lactic acid. There are now techniques to split the lactic acid from its basic salt component and recycle it back to the fermentation chamber, most notably through electrodialysis which creates a LA acidic product stream and a basic waste recycle stream. Constant removal of waste products and maintenance of optimal growing conditions creates a more efficient system with higher productivity.
Continuous run fermentation is more efficient with higher productivity than batch runs by maintaining stationary cell densities, cells do not divide and more sugar consumed is turned into the desired product. Continuous run fermentation is through the removal of product rich media and the addition of fresh nutrients while maintaining cell densities. A standard configuration is to remove the product rich broth, filter the broth through a fine mesh that captures the cells, and return an amount of fresh nutrients equal to the product broth removed to the chamber along with the captured cells. Optimization is needed for different microorganisms, end-product concentration inhibition, and optimal nutrient levels in order to maximize efficiency. A bioreactor with necessary sensors makes this process much more manageable.
Purification of lactic acid
The chemical formula of lactic acid is CH3CHOHCOOH with a single chiral group and two isomer forms (D and L). Isomers are molecules of the same chemical formula that exhibit a difference in positioning of four different chemical groups on a central atom (lactic acid has a carbon with attached methyl, hydroxyl, hydrogen, and carboxyl groups). Isomer composition is important to crystallization with heterogenous mixtures forming amorphous configurations. Purification is aided by single isomer production in the fermentation step.
Membrane based filtration is proposed to be the basis of a sustainable, small scale polylactic acid production process for its relatively low running and capital costs and supposed high recovery and running efficiency. A large degree of information capital must be put into the design of a series of membranes to attain high quality end products with a minimal need to replace membranes. Based upon a review of public domain information from literature and expired patents, a preliminary membrane purification scheme is proposed and open questions posed.
Extensive experimentation has gone into tested different configurations of membrane purification for organic acid purification over the last 25 years and resulted in commercialization over the last 10-15 years. Key to successful implementation is preventing system fouling. A design often separates the fermentation broth first by particle size then charge. Microfiltration to a cell free solution and then nanofiltration to retain unused sugars will be pursued. Recycling of both retentates to the fermentation chamber will result in high efficiency continuous operation without loss of feedstock. It has been found that cross flow microfiltration is the optimal first step that separates cells and prevents fouling, and is optimally configured for cell recycle.
Permeate from the size separation chamber will be passed to an electrodialysis stack of 3-8 cells formed by pairs of anion and cation ion exchange membranes. A circulating solution of NaOH will maintain electrode charges and concentrated streams will be passed to a watersplitting electrodialysis stack. After final concentration (to 90%) the product will be tested for purity and polymerization quality.
Polymerization
Polymerization of lactic acid is through condensation reactions forming an ester bond between the carboxyl group and the methyl group (a H2O is removed by elimination of a hydroxyl from the carboxyl and a hydrogen from the methyl group). The reaction is near thermodynamic equilibrium and can be driven by heat, catalysts, and/or vacuum pressure. There are multiple routes to a PLA product. The initially commercialized route of creating polylactic acid was through catalytic Ring Opening Polymerization (ROP) of dilactides. Procedures conducted ROP over catalysts including tin, aluminium and zinc, and sometimes initiators such as butyl lithium (electron donor?) (Achmad et al).
Recent protocols for direct polymerization have been described. The reaction can proceed under a vacuum and temperatures 150-300 C using distillation equipment to remove formed water. This configuration can be used with or without a catalyst and is an active area of research. High molecular weight polymers are desirable and difficult to produce with direct polymerization. Vacuum dehydration procedures capable of producing high molecular weight species with catalysts have been described. A small scale experiment uses a microspatula of Tin(II) chloride to catalyze 5 ml of lactic acid over a bunsen burner and cobalt chloride paper can be used to test for water vapor indicating the reaction is proceeding.
A literature review has been conducted and the current state of the field has been described. Direct polymerization using a reactor chamber configured for vacuum condensation and catalysts appears feasible on a small scale. SnCl2 or tin octenoate is a proposed first generation catalysts with an appropriate initiator of toluene sulfonic acid. The reaction will be driven by added heat and removal of water under vacuum and with a liquid condensor. A two step reaction is necessary to achieve the high desired molecular weights necessary. The first step would use a tin and TSA catalyst under heat and vacuum, followed by a second round of polymerization using just the TSA under heat and vacuum. A detailed protocol is located on the manufacturing page.
Quality and value adding
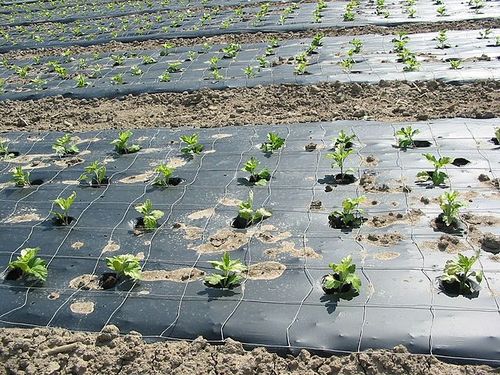
PLA can be recycled with hydrolysis back to monomers.
Plasticizers such as OLLA and polyethylene glycol can improve plastic characteristics.
Process Design
Production of lactic acid monomer
1. Obtaining feedstocks and chosen polylactic acid producing microorganism.
2. Small scale glassware test runs of conditions.
3. Deciding upon protocol for fermentor.
4. Construction of fermentor and subsystems. Construction or purchasing of lactic acid purification equipment (maybe test with small scale runs).
5. Production run of fermentor.
6. Purification of monomer.
7. Demonstrate polymerization of locally produced resource.
Demonstrate small scale catalyst and processing from locally produced or purchased commodity substrate
1. Configure fluidized bed reactor for solid state polymerization under vacuum, no or limited gas feed from below. Alternatively could conduct experiments in glassware.
2. Obtain lactic acid from chemical supplier along with SnCl2 and p-toluenesulfonic acid. Possibly other protonic acids for substitution.
3. Follow melt/solid polycondensation protocol to obtain high molecular weight PLLA.
4. Experiment with plasticizers particularly oligomeric lactic acid and polyethylene glycol which are compatible with OSE product ecologies.
5. Demonstrate thermomoldable PLLA with a range of characteristics from small scale production.
Catalyst and reagents
1. Tin may be available from waste streams or be refinable in the area. Tin chloride is currently used in finishing mirrors as part of that product ecology.
2. Production of higher level organic molecules such as p-toluenesulfonic acid will require further chemical engineering projects.
System Engineering Breakdown Diagrams
Lactic acid production
Polylactic acid polymerization
Design Rationale
The OSE polylactic acid process is designed to conform to OSE standards and utilize open source information to maximize efficiency on a small localized scale. High purity starting substrates will be used to minimize downstream purification machinery. It is an easier task and necessary to demonstrate the ability to produce the end product of the process using commodity materials. Over time resource substitutability and expansion of OS capabilities will allow the production of the materials. A first step is to demonstrate and disseminate the background and process information, and test the feasibility of the end goal.
Catalysts assisted by optimal conditions have high reactivity and result in a high quality product. The current approach proposes to build the machinery necessary for high quality polymerization reactions and demonstrate their use as modular pieces in a bioplastic enterprise. First demonstrations are proposed to use commercial chemical monomers while monomer production and purification is pursued from local feedstocks.
L(+) lactic acid in the form of should be obtained in 90%+. Tin(II) chloride dihydrate and p-toluenesulfonic acid 99%+ should be obtained. Necessary drying, solvent, and vacuum equipment must be obtained. The prototype 1 fluid bed reactor can be configured for a vacuum environment and used to control the reaction. If product is obtained it should be tested with industry measurements and FeF dogfooding. Addition of plasticizers and comonomers can be tested.
Production of substrate monomer lactic acid is proposed through the use the thermophile Bacillus coagulans. Bacillus coagulans 36D1 is a recently characterized homolactic lactic acid bacteria that demonstrates high efficiency. The organism's high optimal temperature of 55 C is advantageous to production runs due to the elimination of the need for autoclaving the feedstock and decreased risk of contamination. Commercial routes currently utilize lactobacillus.
Purification of monomeric lactic acid is proposed to involve dialysis of the lactate salt stream that incrementally introduces semipermeable membranes (first based on size then charge bias). Commercially conventional electrodialysis is used to create a concentrated stream of lactic acid that is further purified and concentrated with water splitting electrodialysis. A polishing step is then used often ion-exchange with a strong acid membrane and weak base membrane. Alternatively, reactive distillation will be used as second purifying step where methanol esterifies with lactic acid to produce methyl lactate. Methyl lactate can be distilled in the bottom of a fraction column and then hydrolyzed to methanol and lactic acid. This two-step purification will have to be tested for its polymerization quality.
Papers
- Review article on LA feedstocks, PLA synthesis, and companies involved. Lactobacillus delbrueckii can ferment table sugar (sucrose). These bacteria need suppliements, best is yeast extract. Starch fermentation can be done with Lactobacillus amylophylus- [2].
- Review of PLA synthesis - [3]
- Source of patented isolated bacillus - [4]. Patent discussing the advantages of this bacillus - [5]. Advantage is that this bacillus isolate can be used with cellulose enzymes in a single vat - avoiding the necessity to otherwise use acids (which also require removal of acid for effective fermentation). The acid route inlvolves acid removal or neutralization - whereas the enzyme + isolate can occur all in the same batch.
- Bacillus coagulans for PLA production from woody biomass. Lactobacillus + sugars are demoted by this paper since it competes with food sources. B. coagulans addresses the issue of culture survival in the presence of acid used to break down the biomass. [6]